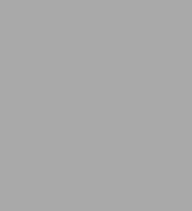
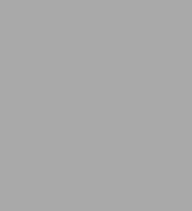
eBook
Available on Compatible NOOK devices, the free NOOK App and in My Digital Library.
Related collections and offers
Overview
The "pedestrian approach" was developed to describe some essentially simple experimental results and their theoretical implications in plain language. In this graduate-level text, Harry J. Lipkin presents simply, but without oversimplification, the aspects of beta decay that can be understood without reference to the formal theory; that is, the reactions that follow directly from conservation laws and elementary quantum mechanics.
The pedestrian treatment is neither a substitute for a complete treatment nor a watered-down version. Its derivation of results obtainable without the formal theory makes these results more understandable and less mysterious to those who have neither the time nor the inclination to master the details of the theory. On the other hand, those already acquainted with the formal theory will find in the pedestrian treatment a clear distinction between results dependent on the specific assumptions underlying the formal theory and those independent of these assumptions, which follow from simple general principles. Since peculiar experimental results are an ever-present possibility, it is always useful to have a simple method of evaluating challenges to theoretical assumptions or approximations.
Product Details
ISBN-13: | 9780486151335 |
---|---|
Publisher: | Dover Publications |
Publication date: | 11/29/2012 |
Series: | Dover Books on Physics |
Sold by: | Barnes & Noble |
Format: | eBook |
Pages: | 128 |
File size: | 3 MB |
Read an Excerpt
BETA DECAY for PEDESTRIANS
By Harry J. Lipkin
Dover Publications, Inc.
Copyright © 2014 Dover Publications, Inc.All rights reserved.
ISBN: 978-0-486-15133-5
CHAPTER 1
INTRODUCTION
1.1. DIRAC MATRICES AND RACAH ALGEBRA
After the discovery that parity was not conserved in beta decay, an extensive series of experiments was undertaken in many laboratories to determine the nature of the beta decay interaction. For a general review of these experiments and a detailed bibliography see KONOPINSKI [1959] and SCHOPPER [1960]. These were mainly measurements of the angular distributions and polarizations of particles emitted in and after beta decay. Many calculations were made giving the results of these experiments as a function of the parameters (coupling constants) characterizing the beta decay interaction. In general these calculations involved complicated manipulations of Dirac matrices and extensive use of the algebraic techniques developed by Racah for coupling angular momenta. On the other hand, it was often possible to obtain considerable insight into the physics of a particular experiment by simple geometrical arguments, usually accompanied by hand waving, drawing pictures, and describing particles as 'spinning this way' (or that way) and going 'up' (or down). Despite the success of these arguments the general feeling prevailed among those in the field that they were 'good for experimentalists', not quite respectable, and that the right way to treat the problem was with the full paraphernalia of Dirac matrices and Racah algebra.
The purpose of this little book is to show that these simple arguments can be put on a rigorous, respectable basis, and to develop them as much as possible. We shall see how much beta decay physics can be described and understood without using Dirac matrices and Racah algebra. Perhaps the reader will feel some of the surprise and delight felt by the author in first learning how far one can go with this simple approach.
The author bears no prejudice against Dirac matrices and Racah algebra. They are interesting, useful, and certainly necessary for a full description of all the aspects of beta decay. However, considerable physical insight is gained by exploring the extent to which the experiments can be understood without Dirac matrices and Racah algebra, particularly in the allowed transitions.
In doing without Dirac matrices and without the Dirac equation, we are essentially doing completely without what is usually called the theory of beta decay. All results obtained in this way, all the relations obtained between the results of various experiments, are therefore independent of conventional beta decay theory. Our treatment will therefore show what kind of experimental information is required to test beta decay theory, and what kind of experimental information is independent of beta decay theory and really tells us only the momentum and angular momentum are conserved.
In doing without Racah algebra we are not really doing anything profound. It simply happens that in allowed beta decay the angular momenta involved are so small (both the electron and neutrino have total angular momentum one-half) that all the appropriate angular momentum coupling coefficients reduce to trivial cases. It is really a shame to use such a powerful and elegant method for such a simple problem. We shall instead present a rigorous treatment of angular momenta which is simply related to the hand-waving picture-drawing arguments.
In the remaining sections of this chapter, we present a summary of the simple picture-drawing arguments, the conclusions which can be inferred, and an analysis of the underlying physical basis. In the following two chapters we develop these arguments into a rigorous theory of beta decay without Dirac matrices, demonstrating all results which are independent of beta decay theory. These results are complete except that the exact explicit form of the angular distributions is not given. In the next two chapters, the necessary properties of angular momentum are developed and applied to obtain exact quantitative results. Finally the predictions of beta decay theory are discussed in the light of the preceding simple treatment without going into the details of the theory, and the present experimental situation is discussed.
1.2. PROPERTIES OF THE ELECTRON AND NEUTRINO EMITTED IN BETA DECAY
In beta decay a nucleus emits an electron and neutrino and is transformed into another nucleus of the same mass number and having a charge differing by one unit from that of the initial nucleus (or a nucleus can capture an orbital electron and emit a neutrino).
[MATHEMATICAL EXPRESSION NOT REPRODUCIBLE IN ASCII]
In beta decay experiments it is generally some properties of the emitted leptons, the electron and neutrino, that are measured, such as their energy, polarization or angular distribution. For this reason it is of interest to examine the possible properties and states of these leptons.
Both the electron and neutrino have spin one-half. There are therefore two possible orientations for each spin. We can describe these two states in several ways. If the motion of the particle in space is described by a plane wave, we can talk about 'spin up' or 'spin down'. If the particle has a well-defined total angular momentum j, we can say that there are two possible states for each value of j with 'opposite' spin orientations, one in which the orbital angular momentum l is j + 1/2, and one in which l is j - 1/2. Since the orbital angular momentum is not a constant of the motion for a relativistic particle, but parity is, we can modify this classification by saying that there are two states for the same j, one with odd parity and one with even parity. In the non-relativistic limit this reduces to the case where the two states have a definite orbital angular momentum. For example, if j = 1/2, then the orbital angular momentum can be zero or one (s-state or p-state). The states of even and odd parity are both mixtures of s1/2 and p1/2 with the degree of mixing depending upon the energy. In the extreme relativistic limit they are both equally mixed; in the non-relativistic limit they are separated with the s-state having even parity and the p-state odd.
In beta decay, angular momentum is conserved but it is now known that parity is not conserved. The leptons are emitted in states of indefinite parity. Thus it is not convenient to use parity to distinguish between the two states of the same angular momentum j. As longitudinal polarizations of particles are frequently measured, a more suitable way to specify the spin direction is in terms of the longitudinal polarization, or helicity. This classification is commonly used with circularly polarized light quanta. We thus have 'left-handed' states in which the direction of the spin is opposite to the direction of motion and 'right-handed' states in which the direction of the spin is parallel to the direction of motion.
In allowed beta decay transitions, the electron and neutrino are both emitted in states of total angular momentum j = 1/2. There are therefore two possible directions for the orientation of the total angular momentum with respect to axes fixed in the laboratory. The projection of j on the fixed axis is usually denoted by m and can take on the values m = ± 1/2.
In allowed decay each lepton has four possible states. It can be either left or right-handed, and its angular momentum can be pointing either 'up' (m = +1/2) or 'down' (m = -1/2). There are thus 4 X 4 or sixteen possible states for the combined electron-neutrino system. These states are represented graphically in Fig. 1.1.
1.3. PICTORIAL PREDICTIONS OF EXPERIMENTAL RESULTS
Fig. 1.1 shows the sixteen possible electron-neutrino states of allowed beta decay. In each diagram the lines indicate the paths of the electron and neutrino emitted from the nucleus; the arrows indicate the direction of the spin. Each path is labeled e or v indicating that the particle is an electron or neutrino. The subscripts R and L indicate that the particle is right-handed or left-handed. The diagrams are also labeled by the projections of the electron and neutrino angular momenta on a vertical axis, me and mv.
Each diagram in Fig. 1.1 indicates a definite direction of emission for each lepton with respect to the vertical axis, and therefore with respect to the direction of emission of the other lepton. This is equivalent to the simple 'hand-waving' arguments like the one that a particle whose angular momentum is directed upward ('it's spinning this way') and which is right-handed, must be moving up. It is a trivial result of the definition of 'right-handed' or 'left-handed' longitudinal polarization (or helicity) as the sense of the projection of the spin on the direction of motion. If any two of these three quantities is known, spin direction, momentum direction, and polarization, the third is uniquely determined by this definition. We can thus express any one of the three quantities in terms of the other two. This trivial result is used extensively in the simple arguments interpreting allowed beta decay experiments.
Let us now examine the three common types of experiments measuring angular distribution in allowed beta decay.
1. Angular distribution of electrons emitted from polarized nuclei. This was the first experiment performed which showed that parity was not conserved in beta decay (Wu [1957]). We take for simplicity the case which was first measured, that of Co60. The spin of Co60 is 5, and it decays by negaton emission to a state of Ni60 which has a spin of 4. The cobalt nuclei are polarized by a magnetic field at low temperature and the angular distribution of the beta rays is measured with respect to the direction of nuclear polarization. If parity were conserved, equal numbers of electrons should be emitted parallel and antiparallel to the direction of nuclear polarization. The asymmetry found experimentally in the angular distribution proved that parity was not conserved in beta decay.
Let us now try to analyze this experiment using simple arguments and the diagrams of Fig. 1.1. For simplicity we assume that the nuclei are completely polarized. We choose the direction of nuclear polarization as our axis of quantization. The Co60 nuclei are therefore initially in a state whose angular momentum has a projection in the direction of our axis of Mi = + 5, as is shown in Fig. 1.2. The final nuclear state has a total spin of 4. Its projection on the axis must be Mf = + 4, as the electron and neutrino can each only carry an angular momentum of , and angular momentum must be conserved. We see that the projections of the electron and neutrino angular momenta on our axis are determined by these considerations, and that the me = mv = +1/2.
Knowing the directions of the lepton angular momenta selects four diagrams out of the sixteen of Fig. 1.1, corresponding to the four possible combinations of directions of polarization of the two particles. From these diagrams, for me = mv = +1/2 in Fig. 1.1, we see that the electron goes 'up' if it is right-handed, or 'down' if it is left-handed, independent of the state of the neutrino. (If the electrons are unpolarized, as would be the case if parity were conserved, then half of them go up and half down, and there is no asymmetry.)
This experiment is thus completely equivalent to a measurement of the degree of longitudinal polarization of the beta particles emitted from Co60. The relation between the results of the angular distribution experiment and the polarization measurement is thus independent of beta decay theory. The results of one of these experiments can only confirm or contradict the information obtained from the results of the other. They cannot give any new additional information about beta decay.
2. Beta-gamma circularly polarized correlation. Let us consider a decay scheme such as is shown in Fig. 1.3 in which a nucleus of spin zero decays by beta emission to a nucleus having spin one, which then decays by gamma emission to a nucleus having spin zero. The gamma ray is detected through a polarization analyzer, such as scattering in magnetized iron, in coincidence with the beta rays. The angular distribution of the beta rays is measured with respect to the direction of emission of the polarized gamma ray.
Let us take as our axis of quantization the direction of the polarization of the gamma ray (in the direction of emission if the gamma ray is right-handed, opposite if it is left-handed). The projection of the gamma ray angular momentum on our axis is thus Mγ = + 1 as shown in Fig. 1.4. Since both the initial and final states of the nuclei in this beta-gamma cascade have spin zero, the total angular momentum carried away by the two leptons and the gamma ray must be zero as well. The projections of the lepton angular momenta on our fixed axis must therefore be equal and opposite to the projection of the gamma ray angular momentum. Thus me = mv = -1/2, as shown in Fig. 1.4.
Now that the directions of the lepton angular momenta are known, we can immediately predict the direction of emission of the electron, as in the previous case. Since we have chosen our axis here so that m = 1/2 instead of +1/2, the predictions are opposite to those of the previous case. The electron goes up if it is left-handed, down if it is right-handed.
We see that this experiment is equivalent to the previous experiment and also measures the degree of longitudinal polarization of the electron.
This result can be expressed more conveniently if we note that the direction of emission of the gamma ray is up, if the gamma ray is right-handed, and down if it is left-handed. This is simply because we have chosen an axis in the direction of polarization of the gamma ray. The relation between the relative directions of emission and polarization of the electron and gamma ray can be summarized as follows: the electron and gamma ray are emitted in opposite directions if the senses of polarization of the two are the same (i.e. both left-handed or both right-handed); they are emitted in the same direction if the senses of polarization of the two are opposite.
The experimental result can be obtained by measuring the difference in the coincidence counting rate for two directions of beta ray emission, with a given direction of gamma ray polarization selected. It can also be obtained by looking at the change in counting rate with reversal of the direction of polarization of the gamma ray selected for a given direction of beta and gamma ray emission. The latter is more practical, since it involves only the reversal of a magnetic field and no changes in geometry.
3. Electron-neutrino angular correlation. Let us consider a case in which the initial and final nuclear states in a beta decay both have spin zero, and the angular correlation between the directions of emission of the electron and neutrino is measured. Since the total angular momentum carried by the leptons must be zero to conserve angular momentum, the components of the two angular momenta on any axis must be equal and opposite. One is +1/2, the other -1/2. The relevant diagrams in Fig. 1.1 are thus eight on the right-hand side of the figure. From the diagrams we see that if both leptons are right-handed, one goes up and the other down, and the same if both are left-handed. On the other hand, if one is left-handed and the other right-handed, they both go in the same direction.
The electron-neutrino angular correlation experiment therefore measures whether the longitudinal polarization or helicity of the electron has the same sense as that of the neutrino, or whether it has the opposite sense.
1.4. THE PHYSICAL BASIS OF THE SIMPLE PICTURE
In the three simple cases discussed above, we have been able to relate the results of angular distribution measurements to the angular momenta and polarizations of the particles in a manner independent of beta decay theory. Although the treatment is oversimplified, the underlying physical basis is valid. The existence of the diagrams of Fig. 1.1 and the selection and interpretation of those diagrams relevant to a particular decay follow directly from simple principles. A rigorous treatment can be based on these same principles to give exact relations between experimentally observed quantities which are independent of beta decay theory.
The essential feature underlying the interpretation of the diagrams of Fig. 1.1 is the simple, almost trivial, relation between momentum, angular momentum and sense of polarization. This interpretation is oversimplified, as is indicated by the representation of leptons as always going either 'up' or 'down'. We know that there is no limitation in practice to these two directions, and that there should really be a continuous distribution. This inadequacy has nothing to do with beta decay theory, it results merely from our improper treatment of angular momenta in a quantum-mechanical problem. This can be rectified by a proper treatment of the angular momenta which gives the exact relations for all angular distributions. Because of the simplicity of the cases relevant in allowed beta decay, Racah algebra is not essential and we shall obtain all angular distributions without its use.
(Continues...)
Excerpted from BETA DECAY for PEDESTRIANS by Harry J. Lipkin. Copyright © 2014 Dover Publications, Inc.. Excerpted by permission of Dover Publications, Inc..
All rights reserved. No part of this excerpt may be reproduced or reprinted without permission in writing from the publisher.
Excerpts are provided by Dial-A-Book Inc. solely for the personal use of visitors to this web site.
Table of Contents
I. IntroductionII. Specification of the Initial and Final States
III. Allowed Beta Decay in Words and Pictures
IV. Angular Momentum in Beta Decay
V. Allowed Beta Decay with Formulas
VI. Connection with Beta Decay Theory--Conclusions