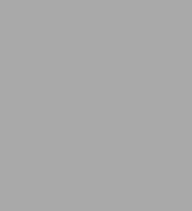
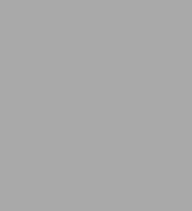
Hardcover
-
PICK UP IN STORECheck Availability at Nearby Stores
Available within 2 business hours
Related collections and offers
Overview
The authors show that global patterns of biodiversity fall into four consistent categories, according to where species live: on land or in coastal, pelagic, and deep ocean habitats. The fact that most species groups, from bacteria to whales, appear to follow similar biogeographic patterns of richness within these habitats points toward some underlying structuring principles. Based on empirical analyses of environmental correlates across these habitats, the authors combine aspects of neutral, metabolic, and niche theory into one unifying framework. Applying it to model terrestrial and marine realms, the authors demonstrate that a relatively simple theory that incorporates temperature and community size as driving variables is able to explain divergent patterns of species richness at a global scale.
Integrating ecological and evolutionary perspectives, A Theory of Global Biodiversity yields surprising insights into the fundamental mechanisms that shape the distribution of life on our planet.
Product Details
ISBN-13: | 9780691154831 |
---|---|
Publisher: | Princeton University Press |
Publication date: | 06/12/2018 |
Series: | Monographs in Population Biology , #60 |
Pages: | 232 |
Product dimensions: | 6.30(w) x 9.30(h) x 0.90(d) |
About the Author
Read an Excerpt
CHAPTER 1
Introduction
The most striking feature of Earth is the existence of life, and the most striking feature of life is its diversity.
— David Tilman
Our planet is characterized by two highly unusual, and intriguingly beautiful, aspects: the fact that it contains life at all, and the spectacular ways in which novel life forms have diversified and assembled into complex communities and ecosystems. We have not — at the time of writing — discovered life on other celestial bodies, and even if life forms do exist elsewhere, the complexity of Earth's diversity may still remain unique or inordinately rare. As far as we know, the first microbial life on Earth originated in a primordial abiotic ocean some 3 to 4 billion years ago, possibly at hydrothermal vents (Martin et al. 2008), although several competing hypotheses exist (Mulkidjanian et al. 2012). From there, the remarkable biodiversity that now defines and shapes life on our planet evolved further, dispersing across the global ocean, and, about 0.6 billion years ago, onto the land (Retallack 2013). A cornucopia of profound biochemical, physiological, structural, and behavioral developments and adaptations facilitated this expansion into previously lifeless and often hostile environments. The evolutionary history of marine to terrestrial colonization is still visible in the distribution of higher taxa today: almost all animal phyla occur in the oceans, while fewer than half exist on land (May 1988). Yet, total species richness of eukaryotes is estimated to be about threefold greater on land (Mora et al. 2011), in large part due to the extraordinary radiation of insects. Remarkably, however, we have only a rough idea how many species exist either on land or in the oceans, possibly comprising some 8 to 9 million eukaryotes in total (Mora et al. 2011), with most of them still awaiting formal description. The challenge of identifying and explaining the seemingly endless variety of life on Earth remains one of the most profound tasks in biology, and perhaps in the sciences as a whole.
Apart from the sheer magnitude of biodiversity, its prominent spatial patterns have long been of interest. The nonrandom spatial distribution of biodiversity is apparent even to the casual observer. It is likely that early hominids who traveled and expanded their reach across different biomes would have already been cognizant of the substantial differences between the richness of ecological communities at large scales and their broad relationship to latitude, altitude, and moisture regimes. Ever since naturalists began describing, organizing, and documenting the variety of species in more detail, some very general patterns have emerged. For many taxonomic groups surveyed on land, including plants, vertebrates, and insects, species richness (the number of species found in a particular area at a given time) peaks in the wet, warm tropics and falls off sharply toward higher latitudes and altitudes (Gaston 2000). This first-order pattern of global biodiversity (Krug et al. 2009) is exceedingly well documented (Hillebrand 2004), but poorly understood from a theoretical perspective, with a raft of possible mechanisms proposed (Rohde 1992). In the oceans, similar gradients in species richness have been observed from the tropics to the poles in some well-studied coastal taxa (Stehli et al. 1967; Roberts et al. 2002). Open-ocean (pelagic) species, from zooplankton to whales, have been analyzed more recently and often display a different pattern that peaks at subtropical or even temperate latitudes (Tittensor et al. 2010). Deep-sea taxa, while sparsely sampled, appear different again (Woolley et al. 2016). Explaining these large-scale patterns has become a core question in ecology and evolutionary biology, often addressed through correlative methods linking hypothesized mechanisms to environmental variables. Although such approaches can help to identify possible drivers, they cannot necessarily distinguish between them or shed light on the mechanisms involved. An alternative route for enhancing our understanding of the spatial distribution of global bio-diversity is through developing a model that enables, or at least proposes, a mechanistic understanding of the fundamental processes structuring global patterns in biodiversity, an ideal that has been called the "holy grail of modern biogeography and macroecology" (Gotelli et al. 2009).
Clearly, fundamental questions about species diversity are not just interesting to ecologists but are also central to our understanding of the world we live in and how we relate to it. Species diversity is akin to a periodic table of biology: it provides the fundamental building blocks for the ecosystems we all inhabit. How is the global richness of species organized, and how does it vary across taxa and through space and time? What are the environmental factors that may drive this variation and to what extent are these factors influenced by human perturbations? And finally, can we explain this bewildering variety from simple ecological theory and provide a more mechanistic understanding of the fundamental distributional patterns of life? These questions have long been at the core of ecology, and they form the focus of this book.
1.1. INTEGRATING LAND AND SEA
A key premise of our work here is that a more comprehensive understanding of global biodiversity can be gained only by overcoming a disciplinary divide that has long separated the study of biodiversity on land and in the sea. Since humans are land animals, we have often been primarily concerned with the terrestrial organisms that we can more easily observe. This is also reflected in the history of ecological study. Many scientists who shaped the foundations of our field, such as von Humboldt, Darwin, Wallace, Elton, Hutchinson, and MacArthur, had a terrestrial or sometimes freshwater focus, but they rarely considered the oceans in detail (Darwin's beautiful monographs on corals and barnacles being among the notable exceptions). These pioneers also shared a keen interest in the forces that shape patterns of species richness. Hence, published work on this topic is heavily biased toward the land. A quick online search confirms that there are about 30% more scientific articles and books devoted to the biodiversity of a single terrestrial habitat type — forests — alone than to marine biodiversity. Likewise, the mention of "plant diversity" in all published books, scientific or otherwise, goes back at least to the beginning of the twentieth century, whereas marine species groups appeared much later in this context and have only slowly risen to more prominence (fig. 1.1).
In focusing on the oceans in addition to the land in this book, we treat different marine habitats as further "replicates" of global biodiversity patterns: different from terrestrial, but potentially organized (at least at some fundamental level) by similar principles (fig. 1.2). At the very least, this assertion is a null-hypothesis to be confronted with data. At the very best, it increases the "degrees of freedom" when we test our ability to understand and predict the first-order patterns of biodiversity on our planet. As the marine environment is less familiar to most, it tends to challenge our assumptions about how the world works, and allows us to critically examine ecological concepts that have been developed with a largely terrestrial focus. Are these patterns, ideas, hypotheses, and theories truly general to all systems? It is our assertion that ecology, and its theoretical foundations, should be general, no matter whether its subjects happen to be wet or dry.
At the same time, our — and indeed society's — current interest in biodiversity is much more than academic. The rapid erosion of biodiversity — both on land and in the ocean — is cause for much concern, as it threatens individual species with extinction (Dirzo et al. 2014; McCauley et al. 2015), and ecosystems with loss of functionality, essential services, and resilience (Worm et al. 2006; Hooper et al. 2012). There is growing awareness of this biodiversity crisis as a defining problem of our time (Tickell 1997), with international policies being shaped in an attempt to slow and ultimately reverse the rate of loss (Tittensor et al. 2014). This has also resulted in renewed interest in the fundamental processes that give rise to, maintain, or threaten, biodiversity at local, regional, and global scales.
Some of these fundamental processes can be further unveiled by contrasting and comparing current patterns of and changes in diversity to those that have unfolded through deep time (Jablonski et al. 2006; Valentine and Jablonski 2015; Yasuhara et al. 2015). By carefully dissecting the paleontological record, researchers have been able to trace patterns of species richness throughout Earth's history, learning how they have changed as the environment around them became altered by geological, biological, and climatic forces (Renema et al. 2008; Krug et al. 2009; Yasuhara et al. 2012; Jablonski et al. 2013; Mannion et al. 2014). At present, human beings are exerting pressures and inducing changes as pronounced as many of these deep-time processes, and are certainly a dominant force shaping life on Earth (Waters et al. 2016). As an example, CO is now emitted at a rate ~20 times greater than the fastest CO emission rates in recorded geological history (Zachos et al. 2008), and is warming the planet at record speed. Yet, our ability to predict the future of biodiversity is both more limited and more uncertain than our understanding of its past (Sala et al. 2000). We posit that a more general understanding of global biodiversity patterns, and in particular the ecological and evolutionary forces that shape them, may help us to forecast biological reconfigurations in both the short and the long term under a given rate and pattern of environmental change.
1.2. A BRIEF HISTORY OF BIODIVERSITY RESEARCH
It is often instructive to trace the origin and evolution of a question or an idea through history. Observations on patterns of species richness at local scales likely date back to the beginnings of human inquiry. Traditional hunter-gatherer societies, for example, acquired and still hold detailed knowledge of many species and their distributions (Cotton 1996), and devised observation-based heuristics to explain such patterns. Early scientific inquiry was exemplified by Aristotle's strikingly detailed observations on both terrestrial and marine animals, particularly their morphology and distribution. These important observations were not synthesized in much greater detail until the Age of the Enlightenment. Specifically, Linnaeus's Systema Naturae (Linnæus 1758) established taxonomy and systematics, and enabled a more structured inquiry into patterns of species richness and how they unfolded based on the relatedness of individual organisms. Subsequently, major scientific expeditions were organized, discovering new species and ecological communities as they surveyed land and ocean areas around the globe. For example, Alexander von Humboldt and Aimé Bonpland's expeditions to South America of 1799–1804 initiated modern biogeography, while the Challenger expedition of 1872–1876 laid the foundations of oceanography, and catalogued over 4000 unknown species from marine waters around the world. A common thread running through this "era of discovery" was that it became increasingly evident that our planet held far more forms of life than had hitherto been anticipated, and that much of it was concentrated in tropical forests on land, or contained in structured habitats such as kelp forests and coral reefs in the ocean. In the marine realm, however, the pace of discovery was generally slower, particularly in the vast reaches of the open ocean and the deep sea, the latter long presumed to be devoid of life.
Darwin's theory of evolution (Darwin 1859) provided another milestone. The theory was elegant in that it required only two fundamental processes — specifically, the generation of variation in species' traits and the forces of natural selection acting upon that variation — to explain the emergence of new species, and by extension all of biodiversity. Although the Modern Synthesis suggests that other processes, such as drift, are also important, the ramifications of Darwin's theory as a structural foundation for anchoring an understanding of the spatial distribution of biodiversity are obvious. Yet, there was little theory available or developed at the time to understand the processes shaping the distribution of those species across regions and around the globe.
Modern biodiversity science was arguably born out of Gene Evelyn Hutchinson's "Homage to Santa Rosalia" (Hutchinson 1959), in which he posed the question of how the great variety of observed species could coexist in a given environment while competing for a few limiting resources. Examples include the astounding richness of plankton species that can coexist in a single drop of water, or the large number of tree species found in a patch of tropical forest. Hutchinson's student Howard Sanders took this question to the deep sea, where he and Robert Hessler discovered surprising levels of macrofaunal diversity that were at the time perceived to perhaps match those of hyperdiverse tropical forests and coral reefs (Hessler and Sanders 1967). Soon Sanders, among others, was formulating theory to explain these observations (Sanders 1968). The stability-time hypothesis, which was borne out of these observations, was one of the first theories specifically developed to explain observed biodiversity patterns; it related large-scale differences in species richness to the severity and frequency of disturbances. This work partially inspired several decades' worth of research into marine biodiversity patterns, both from biologists, focusing on coral reef and deep-sea macrofauna (McCoy and Heck 1975; Grassle and Maciolek 1992; Rex et al. 1993; Roberts et al. 2002; Brandt et al. 2007), and geologists, focusing on bivalves, foraminifera, and other microfossils (Ruddiman 1969; Stehli et al. 1969; Rutherford et al. 1999; Valentine and Jablonski 2015).
In a parallel development, MacArthur and Wilson published their Theory of Island Biogeography, arguably the first mathematical theory of biodiversity patterns (MacArthur and Wilson 1967). While originally focusing on the immigration and extinction of terrestrial species on oceanic islands, it was subsequently applied to other isolated habitats such as lakes, mountaintops, and forest fragments. A later extension of this theory, The Unified Neutral Theory of Biodiversity and Biogeography (Hubbell 2001), which built on MacArthur and Wilson's work, was heavily informed by observations in tropical forests. When its predictions were tested in other habitats both marine (Dornelas et al. 2006) and terrestrial (McGill et al. 2006), however, results were mixed, and its generality remained unclear. The Metabolic Theory of Ecology is another, more recent theory that makes general predictions about changes in community structure and consequently biodiversity in relation to temperature (Brown et al. 2004). On its own, it also falls short of capturing a majority of diversity gradients on land (Hawkins et al. 2007), but it has not been evaluated in comprehensive detail for marine environments.
The new millennium brought about an era of large-scale data integration and synthesis, spurred by the growth of macroecology as a prominent subfield in ecology (Brown 1995; Gaston 2000). The First Global Census of Marine Life (2000–2010), for example, built on Fred Grassle's and Jesse Ausubel's bold vision to systematically chart and understand marine biodiversity patterns across all the many different habitats, from shallow reefs to abyssal plains (Ausubel 1999; Grassle and Stocks 1999). Grassle was a student of Sanders, and thus it is conceivable that the vision of a systematic and global marine census may ultimately be traced back through a direct academic lineage to Hutchinson's seminal thoughts on patterns of species diversity. The Census of Marine Life was by far the largest initiative of its kind and certainly invigorated interest in marine biodiversity, both in academia and in the public eye through regular media coverage of its many spectacular and photogenic discoveries. Scientifically, it fostered an interdisciplinary, comparative, and highly collaborative approach that culminated in a series of synthetic papers on the large-scale patterns of species distribution, abundance, and richness across different marine habitats (Tittensor et al. 2010; Block et al. 2011; Mora et al. 2011; Ramirez-Llodra et al. 2011). At the same time, parallel synthetic developments in terrestrial ecology led to similar efforts at empirical synthesis, focusing largely on patterns in plant (Kier et al. 2005; Kreft and Jetz 2007) and vertebrate species richness (Jetz and Rahbek 2002; Grenyer et al. 2006; Jetz and Fine 2012). Finally, the creation of dedicated institutions such as the National Center of Ecological Analysis of Synthesis (NCEAS) spurred the search for general ecological principles to be extracted from these newly documented global patterns. Most recently, online databases such as the Encyclopedia of Life (http://eol.org/); the Map of Life (https://www.mol.org/); the International Union for Conservation of Nature (IUCN) Red List (http://www.iucnredlist.org/); the Global Biodiversity Information Facility (http://www.gbif.org/); and the Ocean Biogeographic Information System (http://www.iobis.org/) have enabled even better integration of biological and environmental data, facilitating further syntheses of observed patterns of species richness and community structure.
(Continues…)
Excerpted from "A Theory of Global Biodiversity"
by .
Copyright © 2018 Princeton University Press.
Excerpted by permission of PRINCETON UNIVERSITY PRESS.
All rights reserved. No part of this excerpt may be reproduced or reprinted without permission in writing from the publisher.
Excerpts are provided by Dial-A-Book Inc. solely for the personal use of visitors to this web site.
Table of Contents
Foreword 5
Overview of Snakes 7
Snakes’ Venom 25
Where Do Snakes Live? 31
Snakes’ Enemies 33
Conservation Measures 34
Snakes in Captivity 35
The Species 37
Snake Classification 38
The Snakes of Europe, North Africa and the Middle East 43
Families Typhlopidae (blind snakes) and Leptotyphlopidae
(slender blind snakes) 44
Family Boidae (boas) 56
Families Colubridae and Lamprophiidae (colubrid snakes) 66
Family Colubridae 67
Sub-family Colubrinae (terrestrial and arboreal colubrids) 69
Sub-family Natricinae (semi-aquatic colubrids) 207
Family Lamprophiidae 224
Sub-family Lamprophiinae (house colubrids) 225
Sub-family Psammophiinae
(Montpellier snakes and allies) 227
Family Elapidae (cobras and sea snakes) 252
Family Viperidae (vipers) 264
Sub-family Viperinae (true vipers) 265
Sub-family Crotalinae (pit vipers) 362
List of Snakes of the Western Palaearctic 366
Recommended References 372
Index of English Names 375
Index of Scientific Names 377
List of Photographers 379
What People are Saying About This
"I have been awaiting such a book for a long time. Worm and Tittensor combine three leading theoretical perspectives in ecology—neutral theory, metabolic theory, and niche theory—into a single, elegantly simple model to explain global patterns of species richness on land and in the sea. Their theory is novel, often surprising, and important in its implications for the future of biodiversity on Earth."—Stephen P. Hubbell, University of California, Los Angeles, and the Smithsonian Tropical Research Institute"An impressive step forward in our quest to understand the patterns of life on this planet. This book will guide future research and inform the actions that we must prioritize to conserve biodiversity."—Cristiana Paşca Palmer, United Nations Assistant Secretary General and Executive Secretary of the Convention on Biological Diversity"This eminently readable book probes the very heart of ecology and evolutionary biology by describing and explaining global patterns of species diversity. A landmark work and must-read for every serious student of living nature."—James Estes, author of Serendipity: An Ecologist’s Quest to Understand Nature"This book is an outstanding contribution to the long-standing effort to account for the diversity of life on Earth. It summarizes the state of the science, presents a wealth of data on biodiversity in the oceans and on land, and develops a compelling new model to explain major patterns and processes. A Theory of Global Biodiversity sets an agenda for the next decade of research."—James H. Brown, coauthor of Biogeography"A Theory of Global Biodiversity examines the age-old issue of what controls diversity at large scales. While the question is often framed as why are there more species in the tropics, this book rightly tackles the more precise and tractable question of why are there more species in some parts of the globe than others."—Brian J. McGill, University of Maine"The pattern of high diversity at the equator and declining diversity at the poles is one that holds true for many taxa, and a universal explanation for this pattern has long been sought by ecologists, naturalists, and evolutionary biologists, among others. This well-written and organized book brings simple mechanistic ecological and evolutionary theory to bear on the problem. There is no question that this is a significant contribution to the field."—Jay Stachowicz, University of California, Davis