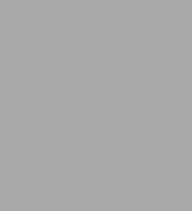
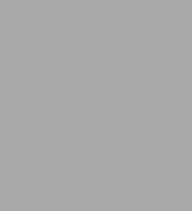
eBook
Available on Compatible NOOK devices, the free NOOK App and in My Digital Library.
Related collections and offers
Overview
"So easy to understand yet so dense with knowledge that you'll never look at waves on a beach the same way again."—San Francisco Chronicle
"One of the best popular science books."—The Kansas City Star
"Perfect for the weekend scientist."—The Richmond News-Leader
A noted physicist and popular science writer heads for the beach to answer common and uncommon questions about the ocean. James S. Trefil, author of Dover Publications' The Moment of Creation: Big Bang Physics from Before the First Millisecond to the Present Universe, explains why the sea is salty, how bubbles form on the water's surface, where waves come from, and other curiosities of the marine world. Dr. Trefil draws upon his unique gift for clarifying complex scientific principles and the theories behind them to present a narrative that is clear, accessible, and utterly compelling.
Product Details
ISBN-13: | 9780486150000 |
---|---|
Publisher: | Dover Publications |
Publication date: | 11/15/2012 |
Sold by: | Barnes & Noble |
Format: | eBook |
Pages: | 224 |
File size: | 9 MB |
Read an Excerpt
A Scientist at the Seashore
By James Trefil, Gloria Walters
Dover Publications, Inc.
Copyright © 1984 James TrefilAll rights reserved.
ISBN: 978-0-486-15000-0
CHAPTER 1
LOOK AT ALL THAT WATER
Little drops of water
Little grains of sand
Make the mighty ocean
And the pleasant land
—JULIA CARNEY, "Little Things"
Stand at the edge of your favorite beach and look out. You are seeing one of the most unusual sights our universe has to offer: large amounts of liquid water. Furthermore, you are looking at water that has existed in a form not too different from its present state for billions of years.
This perception of the oceans of earth as a unique phenomenon is fairly new. Those who read science fiction will have vivid memories of the "canals of Mars" and the "swamps of Venus." Less than a quarter century ago the best scientific guess as to the nature of our planetary neighbors presupposed the presence of large amounts of water. On Mars, the white polar caps indicated that the temperature might be too cold for the water to be liquid, so that it was thought to be locked in ice sheets. On Venus, the cloud cover prevented us from seeing the surface, but it didn't prevent us from imagining the planet as an overgrown version of the Florida Everglades. In both cases, our view of neighboring planets was shaped by the expectation that water, so plentiful on the earth, must be plentiful everywhere else in the solar system.
The planetary exploration program has changed many things about the way we view different environments in the solar system. The new concept of "spaceship earth" has made revolutionary changes in our view of the earth, and brought us closer to a sense of the unique privilege of being earthlings.
Following the Copernican revolution, man has learned to view the earth as a planet—one of many circling the sun. Sir Isaac Newton developed a picture of the universe in which the earth was shaped and governed by the same laws of physics that prevail everywhere. It thus comes as something of a surprise for us to realize that although the same laws operate on earth as elsewhere, those laws have produced a planet which is radically different from its neighbors. Nowhere is this difference more glaring than in the existence of oceans.
The Viking missions to Mars sent back photographs of an arid, lifeless surface; the polar caps turned out to be frozen carbon dioxide (dry ice) Tests showed nothing that couldn't be explained on the basis of ordinary chemical reactions—if there is water there, it must be buried deep underground. Similarly, Venus, with its surface temperature of 800° Fahrenheit, is now known to be much too hot to sustain liquid water. Of all of the planets in our solar system, only the earth has oceans. When the human race begins to move away from the surface of the earth and the first permanent extraterrestrial habitations are built in the next century, the age-old balm of a quiet walk along the seashore may become a thing of the past for our spacefaring descendants.
So the oceans of earth present us with a puzzle. Given that the same laws of nature operate everywhere in the solar system, what is so special about our planet that only we are privileged to have large bodies of liquid water on our planetary surface? What are the unique characteristics which make the earth so different from the other planets, even though the same laws operate here as elsewhere?
To resolve this question, we have to deal with two separate issues: How did the earth acquire such a large amount of water in the first place; and, once acquired, how was the water retained? The first of these questions requires an understanding of how the earth was formed; the second involves the evolution of the earth and its atmosphere.
We know that the planets and the sun were formed in a single process in which a cloud of rotating gas slowly contracted under the influence of its own gravitational attraction. As might be expected from this picture, the largest body in the solar system must be at the center, where the greatest amount of gas collected. This body is the star we call the sun. The heat generated by the collapsing gas eventually became so great at the sun's core that the nuclear fusion reaction was ignited. Hydrogen nuclei were forced together to form helium, and the energy released in this reaction, streaming outward from the core, temporarily stabilized the sun against further collapse. This "temporary" stabilization has lasted about 4.5 billion years so far, and will go on for about 5.5 billion years into the future, when the sun will have burned up all of the fuel that was gathered together in the initial contraction. At that point the sun will die.
Outside of the sun, another process was occurring in the solar system—a process that we still do not understand in great detail. Clumps of gas that had been rotating too fast to fall into the proto-sun and that were therefore left in orbit began to combine to form aggregates through the same gravitational mechanism that had formed the sun. At distances from the sun that now characterize the orbits of the planets, such clumps of gas began to collect. As far as we can tell, this process was random. Somewhere in the thin rotating cloud small clumps of material happened to find themselves close together. The enhanced gravitational attraction of this collection attracted still more material to the spot, which in turn made the gravitational force even greater. It's not hard to see that the process would eventually result in most of the mass in a given area being concentrated in one spot.
It used to be thought that this was how the earth was formed: a random event initiated a collapse until all of the available matter had been swept into a single body. In the last decade, however, this picture has been refined. The best available theories now describe the process of planet formation as having two steps. First, the gravitational collapse we've just described took place, forming small, asteroid-like bodies known as planetesimals—some as large as 1/500th the mass of the earth. The planetesimals then began to collide and combine to form the larger bodies of the planets. The rain of bodies on the surface of the growing earth generated large amounts of heat, enough to cause heavier elements, such as iron, to migrate to the center. In this sense, the earth was actually born with an iron core.
When the planet was still quite small, another interesting physical process started to occur. When a meteor hits anyting, some material sticks and some is scattered back into space by the impact. Of this scattered material, some is moving fast enough to go back into orbit and some is eventually pulled back to the planet. The lower the density of the material, the more likely it is to escape. Thus, in the early stages of its formation, the earth collected heavy material, leaving lighter stuff (such as silicon and water) still in orbit around the sun.
As the earth began to approach its present size, however, the ever-growing gravitational attraction made it harder and harder for debris to escape. By this time, most of the material in the vicinity of the earth's orbit consisted of debris of previous collections and was therefore composed largely of lighter elements. The earth swept through this material, picking up mass the way the windshield of a moving car picks up insects on a summer afternoon. The silicon that makes up the sand on the beach, as well as the water that makes up the ocean, were added to the earth during this last phase of accretion. Each bit of sand or water that you see, therefore, has probably been in space more than once during its history.
The formation of the earth from planetesimals and the sun from the bulk of the original gas cloud took place simultaneously and required a few hundred million years to be completed. When the earth was forming, the fusion reaction in the sun ignited. It was not a smooth process, and can be likened to starting a car engine on a cold morning. The sun stuttered, balked, and backfired for a while—a situation astronomers describe as the T Tauri stage in stellar evolution. Each flaring up of the sun sent strong streams of particles rushing out. These particles swept the remaining gases out of the inner solar system, leaving it in much its present state. Had the earth been formed with a readymade atmosphere present, it would have been blown off at this time, leaving the early earth as a rocky ball with neither air nor water on its surface.
But if all of the water in the atmosphere had been blown out of the solar system by the sun's flare-up, where could the oceans have come from? The answer, paradoxical as it may seem, is that they came from inside the earth. The outer layer of the planet was rich in light materials, including water which had been swept up. The new world was a turbulent place, with volcanoes and earthquakes and other types of what we now call tectonic activity. Each time a volcano erupted or a geyser went off, material from the interior (primarily water vapor but including all sorts of gases) entered the new atmosphere. The process of releasing volatiles in this way is called degassing. Over a relatively short time—100 million years or so—enough volatile material had been released in this way to form the oceans and to give the earth an atmosphere. It wasn't an atmosphere like our own; there was almost no free oxygen, for one thing. But it was a collection of gases which were held at the planetary surface by gravity and which therefore deserves the title of an atmosphere.
Early in this history, perhaps almost from the beginning, the temperature of the earth fell below 212° Fahrenheit and the water condensed into oceans as we know them today. This is not to say that our present oceans formed 4.5 billion years ago—far from it. It simply implies that the large amounts of water we associate with an ocean were on the scene within a few hundred million years of the earth's creation. As we shall see in the next chapter, the present ocean structure is fairly new, the Atlantic Ocean being only 165 million years old.
The weight of geological evidence points to another amazing fact about the early oceans. The amount of water contained within the oceans has not changed appreciably since they were formed. The mass of water in the oceans now (about 1024 gm) is roughly the same as the mass of water that was contained in the crust of the earth when the degassing started. Furthermore, we can estimate the rate at which water is being lost today. The water molecule is too heavy to escape the earth's gravitational pull easily, but water molecules in the air are occasionally dissociated (broken up into the constituent hydrogen and oxygen). The hydrogen freed in this way is light enough to move off into space, which is why there is so little free hydrogen in the earth's atmosphere. The net effect of hydrogen loss, then, is to decrease the amount of water vapor in the atmosphere—water vapor which is replaced by evaporation from the oceans.
About 5 × 1011 gm of water are lost this way each year. This corresponds to a cube 100 yards to a side—about the volume of a small lake. All the water lost to space since the beginning of the earth amounts to about 2 × 1021 gm—less than 0. 2 percent of the water in the oceans. In fact, all of the water lost to space since the beginning corresponds to a square of ocean about eight hundred miles on a side. This means that most of the water you see when you stand on the beach is, in fact, the very same stuff that was degassed from the interior when the earth was only a few hundred million years old.
The small amount of water lost to space is replaced by the same geological processes that formed the present ocean basins. In the next chapter I shall discuss the process by which new ocean floor is created by material moving up to the surface, pushing the old floor aside. This upwelling material contains some water, which, when added to the oceans, balances the loss to space. Geologists call this material added to the oceans "juvenile water." (When I see a scientific term as marvelous as this, I weep for my own field, elementary particle physics, whose contributions include terms like "quark" and misnomers like "strangeness" and "color." For more on this, see my book From Atoms to Quarks.)
In any case, the balance between water lost to space and juvenile water added from the interior guarantees that the total amount of water on the earth's surface must be roughtly constant over long periods of time. It does not, however, mean that all that water must be in liquid form. During the ice ages, an appreciable portion of the water now in the oceans was locked up in glaciers. If there is a fixed amount of water on the surface, then it follows that glaciers can grow only at the expense of the oceans. Thus, when ice covered most of North America and Europe eighteen thousand years ago, the ocean levels were lower than they are now. Large portions of the North Sea and the Baltic Sea were dry land, and Britain was attached to mainland Europe. The east coast of the United States was a hundred miles farther east than it is now, and what is currently part of the ocean floor was populated by land animals (including man).
In a similar vein, the present coastlines of the world are where they are because some of the water supply is tied up in the polar ice caps, mainly on Antarctica. Should the ice caps melt so that all of the water flowed into the ocean basins, the sea level would increase by several hundred feet and flood most of the low-lying coastal areas of the world. But in any case, the fact that your favorite beach is where it is and not a few hundred miles out to sea (or inland) is the result of the way the earth's total water supply is divided between the liquid in the ocean and the solid in glacier and ice cap.
Having found the origins of the ocean in the processes that formed the earth, we can turn to the second (and more difficult) problem we posed earlier. Given that there was enough water to form the oceans early in the earth's history, how is it that the water is still here now, some four billion years later? Presumably the scheme outlined above, in which a planet degasses after its formation, could have happened on any of our neighbors in the solar system, including the moon. Why, then, has the earth alone retained its oceans?
The case of the moon is easy to understand. Because of its light mass, atoms in the moon's early atmosphere had an easy time escaping into space. Long ago they slipped off, leaving the surface exposed to vacuum as it is today. The case of the planets is a little more complicated, so that to understand the present state of the earth we must turn to the remarkable concept of the "continuously habitable zone" (CHZ) introduced by astrophysicist Michael Hart in 1978.
But first we have to realize that during the lifetime of the earth a number of major changes have taken place in the environment, any one of which would be potentially disastrous for the continued existence of the ocean. To begin with, the luminosity of the sun has increased by about twenty-five percent between the time the earth was formed and the present. Had this happened without any compensating changes in the atmosphere, the oceans would have boiled away long ago. Similarly, about two billion years ago oxygen produced by photosynthesis in algae in the earth's oceans started to accumulate in the atmosphere. The oxygen destroyed several gases that were present in the atmosphere by reacting chemically with them. The most important of these were ammonia and methane. Both of these compounds contributed to the greenhouse effect—they were part of the atmospheric "blanket" that kept the earth warm. Removing them was like tearing holes in that blanket; heat started streaming out and the earth cooled off rapidly—almost catastrophically, in fact. Had the cooling been just a little more violent and the energy coming in from the sun just a little less strong, the earth would have frozen solid as soon as a significant amount of oxygen had accumulated in the atmosphere.
What Hart found was that there is a very narrow band around the sun—a band which he called the CHZ—in which it is possible for a planet to tread the narrow line between boiling and freezing. Venus, for example, probably never had an ocean because it is so close to the sun. The atmosphere formed by degassing, acting through the greenhouse effect, kept the planet so warm that the temperature never dropped to the point where the water could condense. New gases added to the atmosphere simply stayed there, increasing the blanket effect and raising the surface temperature still higher. Mars, on the other hand, presents the opposite picture. Because of its small mass and great distance from the sun, whatever liquid water it once may have had has long since either escaped or frozen, leaving the arid ball we see today.
(Continues...)
Excerpted from A Scientist at the Seashore by James Trefil, Gloria Walters. Copyright © 1984 James Trefil. Excerpted by permission of Dover Publications, Inc..
All rights reserved. No part of this excerpt may be reproduced or reprinted without permission in writing from the publisher.
Excerpts are provided by Dial-A-Book Inc. solely for the personal use of visitors to this web site.
Table of Contents
Introduction1 Look at All That Water
2 The Salt Salt Sea
3 The Tides
4 The Search for Planet X
5 The Back of the Moon
6 Making Waves
7 The Surf
8 Bubbles in the Foam
9 The Shape of the Wave
10 Queen Anne's Pudding
11 Of Beaches and Baseballs
12 Castles in the Sand
13 Sailing Ships and Skeletons
14 Skipping Stones
Index