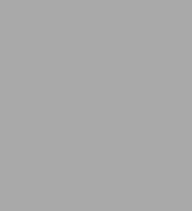
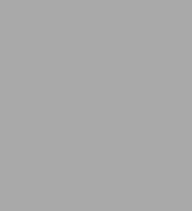
eBook
Available on Compatible NOOK devices, the free NOOK App and in My Digital Library.
Related collections and offers
Overview
Product Details
ISBN-13: | 9780786724017 |
---|---|
Publisher: | Basic Books |
Publication date: | 08/04/2008 |
Sold by: | Hachette Digital, Inc. |
Format: | eBook |
Pages: | 176 |
Sales rank: | 905,652 |
Lexile: | 1270L (what's this?) |
File size: | 253 KB |
Age Range: | 13 - 18 Years |
About the Author
Weinberg, who received his PhD in biology from the Massachusetts Institute of Technology in 1969, has held research positions at the Weizmann Institute and the Salk Institute. In 1982, Weinberg helped found Whitehead Institute, joined the faculty as a professor of biology at MIT, and published his landmark paper "Mechanism of Activation of a Human Oncogene" in the journal Nature. In 1999, another major paper, "Creation of Human Tumor Cells with Defined Genetic Elements," was also published in Nature.
Read an Excerpt
Chapter One
THE ENEMY WITHIN:
GENES, CELLS, AND
THE NATURE OF CANCER
Cancer wreaks havoc in almost every part of the human body. Tumors strike the brain and the gut, muscles and bones. Some grow slowly; others are more aggressive and expand quickly. Their presence in human tissues signals chaos and a breakdown of normal function. Cancer brings unwelcome change to a biological machine that is perfect, marvelously beautiful, and complex beyond measure. Wherever tumors appear, they take on the appearance of alien life forms, invaders that enter the body through stealth and begin their programs of destruction from within. But appearances deceive: The truth is much more subtle and endlessly interesting.
Tumors are not foreign invaders. They arise from the same material used by the body to construct its own tissues. Tumors use the same components--human cells--to form the jumbled masses that disrupt biological order and function and, if left unchecked, to bring the whole complex, life-sustaining edifice that is the human body crashing down.
How are human tissues put together from single cells? The description above might suggest the involvement of master builders who oversee crews of workers, directing them in the detailed construction of normal and malignant tissues. In reality, there are no overseers forcing throngs of cells to line up and assemble themselves into normal or cancerous tissues. Architectural complexity in living tissue comes from the bricks themselves, the individual cells. Control is exercised from the bottom up.
Normal and malignant cells know how to build. Each carries its own agenda that tells it when it should grow and divide and how it should aggregate with other cells to create organs and tissues. Our bodies are nothing more than highly complex societies of rather autonomous cells, each retaining many of the attributes of a fully independent organism.
Right there, we confront great beauty and profound danger. The beauty lies in the coordinated behavior of so many cells to create the single, highly functional cooperative that is the human body. The danger lies in the absence of a single overseeing master builder, which seems to put the whole enterprise at great risk. Granting autonomy to trillions of worker cells invites chaos. When, as usually happens, these cells are well behaved and public-spirited, extraordinarily complex order ensues. But on occasion, a cell may choose to go its own way and invent its own novel version of a tissue or organ. It is then that we see the much-feared chaos that we call cancer.
Most human tumors comprise a billion or more cells before we become aware of them. The cells within a tumor differ from their normal counterparts in many respects, exhibiting distinctive shapes, growth properties, and metabolism. The sudden appearance of such a horde of cells would seem to reflect some recent mass conversion, in which millions of normal cells enlisted overnight in the ranks of a tumor mass.
Once again, appearances deceive. The creation of a tumor is an extraordinarily slow process, often extending over decades. The cells forming a tumor are all lineal descendants of a single progenitor, a distant ancestor that lived many years before the tumor mass became apparent. This founder, this renegade cell, decided to go off on its own, to begin its own growth program within one of the body's tissues. Thereafter, its proliferation was controlled by its own internal agenda rather than the needs of the community of cells around it.
So there were no millions of recruits, only a single one that spawned a vast horde of like-minded descendants. The billions of cells in a tumor are cast in the image of their renegade ancestor. They have no interest in the well-being of the tissue and organism around them. Like the founder cell, they have only one program in mind: more growth, more replicas of themselves, unlimited expansion.
The chaos they create makes it clear how very dangerous it is to entrust each cell in the human body with its own measure of independence. Still, that is how we are put together, and how all complex, many-celled organisms have been designed for the past 600 million years. Learning this, we realize that the chaos of cancer is not a modern affliction but a risk run by all multicellular organisms, from ancient to modern. Indeed, given the trillions of cells in the human body, is it not a wonder that cancer does not erupt often during our long lives?
THE INTERNAL BLUEPRINT
To understand how a tumor grows, we need to understand the cells that form it. What caused that single founding cell to run amok? More generally, how does any cell, normal or malignant, know when to grow? Do cells have their own minds? And if not, what complex decision-making apparatus inside the living human cell determines its growth, quiescence, or death?
Our focus in this book is on the internal program carried by each normal human cell that tells it how and when to grow and associate with other cells to create the highly functional communities that are human tissues. The programs carried by various cells represent complex biological scripts, blueprints for their behavior. As we shall see, it is this internal program that is altered when cancer begins. Only after we understand this program in its normal and damaged forms can we understand the engine that drives the cancer cell.
There are several hundred types of cells in the human body. These various cell types aggregate to form distinct tissues and organs. Knowing this variability of individual cells, we might imagine the existence of a correspondingly large number of distinct scripts, each carried by a different cell type, each dictating a distinct agenda of growth and tissue-forming abilities. Here our intuition leads us astray. In truth, the cells in different parts of our body--in the brain, muscles, liver, and kidneys--as different as they appear, are really very much alike and, unexpectedly, all carry the same blueprint.
This sameness can be traced to their common origin. Like the cells in a tumor, all the cells that constitute a normal body descend from a common ancestral cell. They are cousins, members of an extended family. Through repeated rounds of growth and division, the single cell that is the fertilized egg yields the trillions of cells that form a complete body. The number of cells in an adult human--more than ten thousand billion--vastly exceeds the mind's ability to grasp.
The blueprint that directs all cells in the body is present in the ancestral fertilized egg and is then passed on, virtually unaltered, to all the descendant cells throughout the body. These trillions of cells can look and act very differently from one another, yet they all carry the same set of instructions that program their behavior. So there is a striking discrepancy between the cells' common inner blueprint and their highly diverse outer appearances. It seems that appearance tells us little about the internal program that guides the lives of these cells.
How can a single, common blueprint generate such diversity? Over the past decades the answer has emerged, and it is a simple one: The complex master plan carried by all cells in the body bears far more information than any single cell can use. Each cell in the body consults its master blueprint selectively, reading out only certain information from the vast library that it carries. This information is then used to choreograph its behavior. Selective reading allows each cell to behave differently from its relatives, close and distant, throughout the body.
Shortly after an egg is fertilized, it divides, as do its two daughters in turn. The embryonic development that follows is a frenzy of cell growth and division. The descendants of a fertilized egg arising over the next several cell generations seem very much alike; they stick together tightly, forming a homogeneous, undifferentiated cluster of cells that resembles a tiny raspberry. As embryonic development proceeds, however, the progeny of these cells begin to show differences. They start to become members of the communities of muscle cells or brain cells or blood cells. This process of choosing distinct fates--the process of differentiation--is the central mystery of human development and the obsession of those who study it.
A cell in one corner of the embryo reads out genetic instructions on how to make hemoglobin and becomes a red blood cell; a cell elsewhere consults information on the making of digestive enzymes and becomes part of the pancreas; a third cell reads the information on how to emit electrical signals and forms part of the brain.
But this decision by each embryonic cell to assume distinctive, differentiated traits, reached through selective reading of the genes that it carries, is not the only important decision the cell must make. It must also consult its genetic blueprint on another, equally weighty issue: when it should grow and divide, and when it should stop growing.
These instructions about growth remain important long after the embryonic stage. In most adult tissues, cells are continually dying and being replaced. Indeed, the ability of an adult tissue to maintain its normal architecture is critically dependent on mechanisms that ensure that the occasional loss of cells is compensated by the regrowth of a number of replacements. If too little replacement occurs, the tissue will deteriorate and shrivel away. If there is too much, it will expand, push against its normal boundaries, and perhaps erupt into a tumor. Proper control of cell proliferation is critical throughout the life of an organism.
To understand cancer, we must understand how the inner blueprint of normal cells tells them when they should multiply. We must know how that blueprint becomes deranged in the heart of the cancer cell. The roots of cancer lie in that blueprint.
GENES AND MOLECULES:
A BRIEF PRIMER
The notion of blueprints implies precision, exactitude, a lack of ambiguity. Chaos can be warded off by carefully drawn blueprints. Long before biologists knew much about the inner machinery of living cells, they realized that such blueprints must exist. At first, blueprints were associated with whole organisms; only later did their importance to the lives of individual cells become apparent.
Gregor Mendel, an Austrian monk, established the principle of organismic heredity in the mid-nineteenth century. He focused on the transmission of genetic traits in pea plants--traits such as flower color and seed shape. His work was forgotten and then rediscovered by three geneticists in the first years of the new century. Mendelian genetics, as it came to be called, rested on several simple concepts. First, all complex organisms, from the pea plant to man, transmitted genes from parent to offspring through identical hereditary mechanisms. Second, the outward appearance of an organism could, in principle, be dissected into a large collection of discrete traits, such as flower color and seed shape in peas, eye color or body height in humans. Third, each of these traits could be traced back to the workings of some invisible information packets that were passed from parent to offspring through sexual reproduction. The efficient transmission of these information packets ensured that offspring developed traits closely resembling those of their parents.
The information packets came to be called genes; each human gene was assigned the role of organizing a distinct body trait. As we learned more and more about genes, it became apparent that all aspects of the human body down to the invisible inner workings of individual cells are dictated by the genes that a person inherits from his or her parents. The master blueprint, it turns out, is no more than a large collection of these genes.
We learned that the blueprinting genes are not stored away in a single central archive located somewhere in the body. Instead, almost every one of the trillions of cells in the body carries a complete copy of the entire blueprint. That simple fact reoriented thinking on how genes organize the construction of complex organisms: Genes act directly to control the behavior of individual cells. Each cell, operating under the control of its genes, collaborates with all the others to create the body's form and function. Hence, the complexity of an entire organism represents nothing more than the aggregate behavior of all of its individual cells. This means that the set of genes governing the lives of cells and the set controlling the shape and behavior of the body are one and the same.
A debate of long standing has swirled around the question of how many distinct information packets--individual genes--make up the human genetic blueprint. The best current estimates range between 70,000 and 100,000. Together, these genes form the genetic library, the master blueprint that is commonly called the human genome.
The fact that the genomic library is segmented into discrete gene compartments has several consequences. As mentioned before, different volumes--distinct genes--can be pulled from its genomic library shelf and read selectively by a cell. In addition, these information packets can become separated from one another as they are passed from a parent organism to its offspring. This helps explain why we inherit only some of the genes carried by each of our parents. The genomic libraries in the fertilized egg become a blend of the genes that both parents carried before.
Still, the depiction of genes as information packets is ultimately unsatisfactory, because this image lacks physical reality. Sooner or later, we must deal with the physical substance of genes. Like all other components of living organisms, genes are concrete objects and therefore must be embodied in discrete, identifiable molecules.
Since 1944, we have known that the physical manifestation of genes is the molecule of DNA. Genetic information is carried in DNA molecules. Their structure is quite simple: Each DNA molecule is a double helix composed of two intertwined strands. Each of these strands is a long polymer formed by stringing together, end-to-end, the single components that, for the sake of this discussion, can be termed bases.
The bases of DNA come in four chemical flavors--A, C, G, and T. Importantly, they can be strung together in any order. The information content of DNA is determined by the sequence of these bases. The stringing together of bases can proceed without limit, resulting in DNA strands that can be tens of millions of bases long. A snapshot of one segment of such a very long strand would reveal a specific sequence of bases such as ACCGGTCAAGTTTCAGAG. Modern gene technology allows us to determine these base sequences, a process called "DNA sequencing." By now, several tens of millions of base sequences have been ascertained for various organisms ranging from bacteria to worms to flies to Homo sapiens.
The total flexibility in the ordering of bases in the DNA means that, in principle, any information, biological or otherwise, can be encoded in DNA molecules. At first glance, an alphabet of only four letters would seem limited in its information-carrying capacities. But in fact four letters are more than sufficient. Morse code, with its three letters (dots, dashes, and spaces), and computer binary code with its two-letter alphabet (0 and 1) also have infinite information-carrying capacity.
The double helix of DNA actually carries two copies of genetic information, one on each of its intertwined strands. Since the time of James Watson and Francis Crick's watershed discovery of 1953, we realize that an A appearing in one strand of the helix always faces a T in the opposite strand; a C in one strand inevitably confronts a G in the other. So the sequence of ACCGGTCAA in one strand will be intertwined with a partner sequence, TGGCCAGTT, in the other.
Since the base sequence of one strand dictates the sequence in the other, the information carried in one strand is present in the other, albeit in complementary language. This redundancy has many advantages. Among the most important is that it enables the helix to be replicated. In particular, the two segments depicted in Figure 1.1 can be separated and each can be used as a template for copying a new complementary sequence that then becomes wrapped around its template. The result is two daughter double helices that are identical to each other and to the mother double helix from which they arose.
This copying of base sequences becomes important when a cell grows and divides. During the process, a mother cell prepares to endow each of its future daughters with exact replicas of the DNA helices that it carries. This mother-to-daughter transmission allows the genetic information initially present in the DNA of a fertilized egg to be transmitted faithfully through a succession of hundreds of rounds of cell division to all of the trillions of descendant cells that form the adult human body.
Precisely how does the abstract concept of a gene relate to the physical structure of DNA molecules? The double helices of DNA, carried in the chromosomes of the cell, are often hundreds of millions of bases long. These extraordinarily long strings of bases are divided into discrete segments, in effect information compartments, each compartment constituting a single gene. An average human gene takes up several tens of thousands of DNA bases. Certain punctuation marks, written in the four-letter code of base sequences, demarcate the ends of the gene. In an English text, the beginning of a sentence is marked by a space followed by a capitalized word; the beginning of a gene is indicated by a specific short sequence of tens to hundreds of bases. Similarly, like the end of an English sentence, which is set off by a period, the terminus of a gene has its own distinctive base sequence that serves as a clear punctuation mark. Usually, the end of one gene is followed by a sequence of many thousands of bases of meaningless genetic noise before other punctuation sequences signal the beginning of the next gene along the helix.
The total information content of the human genome is carried in about 3 billion bases of DNA sequence divided into the 70,000 to 100,000 segments that represent individual genes. Somehow these genes, working in various combinations inside our cells, are able to create the extraordinary structural complexity of the human body including that organ of infinite complexity, the brain.
This tale of genes, DNA helices, and base sequences provides an entree into understanding all types of human biology, indeed all life forms on earth. But we are concerned here with a narrow slice of this complexity, the disease of human cancer. We can ignore the difficult question of how genes tell cells to collaborate in constructing tissues and organisms, and focus instead on the narrower question of how genes affect the growth behavior of individual cells.
So we narrow our perspective to address the small subset of genes that tell individual cells whether or not they should grow. These genes take us straight to the heart of the cancer problem. They reveal the origins of cancer and will one day point the way to curing the disease.
Table of Contents
Acknowledgments | v |
1 The Enemy Within: Genes, Cells, and the Nature of Cancer | 1 |
2 Clues to Cancer's Origins: How the Outside World Affects | |
the Insides of Our Cells | 13 |
3 The Elusive Quarry: Hunting the Proto-oncogene | 25 |
4 Fatal Flaws: Finding Oncogenes in Human Tumors | 37 |
5 A Book of Many Chapters: Multistep Tumor Development | 45 |
6 Fueling the Fire: Carcinogens That Aren't Mutagens | 55 |
7 Brake Linings: The Discovery of Tumor Suppressor Genes | 63 |
8 Guts: A Case Study in Cancer Development | 79 |
9 Defenders of the Text: DNA Repair and Its Breakdown | 87 |
10 Guide Proteins of the Cell: The Machinery That Controls | |
Growth | 95 |
11 A Breakdown of Order: Subverting Normal Growth Control | 105 |
12 Immortality: Avoidance of Almost Certain Death | 113 |
13 Assisted Suicide: Apoptosis and the Death Program | 121 |
14 A Clock Without Hands: TheCell Cycle Clock Apparatus | 131 |
15 Many Obstacles in the Road: The Evolution of a Tumor | 141 |
16 An End to the Scourge: Using the Knowledge of Cancer's | |
Causes to Develop New Cures | 151 |
Index | 165 |